“If the bulb dies, the patient dies.”
While perhaps a bit overdramatic, this statement contains a kernel of truth. It emphasizes the well known practical limitations of 300 and 400 W xenon arc lamps in intraoperative imaging applications. It is the main motivation for the replacement of arc lamps with solid-state illuminators. The overriding requirement for clinical applications is the light output delivered to the surgical site must be invariant in terms of radiant and spectral intensity for the duration of the operation, which may be several hours.
Lumencor pioneered the replacement of xenon bulb illumination with solid-state lamps for endoscopy and robotic surgery almost a decade ago. [1] It is widely accepted that the endoscope improves access and visualization through small surgical corridors, while the exoscope is best suited to the demands of large surgical corridors. The exoscope supports improved focal distance, surgeon posture, trainee education, compactness, and assistant participation. Compared to microscopes, exoscopes provide longer working distance, higher magnification, and wider fields of view at a greater field depth, while also providing heads-up visualization for the surgeon and surgical assistants (Figure 1). Solid-state illumination by the exoscope permits reduction in thermal damage and tissue glare that can occur with the conventional microscope. [2,3]
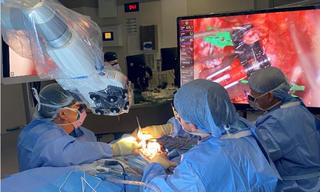
Figure 1. A surgical exoscope in use. The exoscope, mounted on a robotic arm, is positioned between the surgeons and above the patient, projecting white light onto the surgical site below. The monitor on the upper right of the photo displays the magnified image of surgical site.
The spectral output of a SPECTRA Light Engine configured for use in a surgical exoscope is shown in Figure 2. The output is generated from integrated outputs of a proprietary luminescent light pipe source (Green, Figure 2) and 5 LED sources. The spectral output contains a wealth of light throughout the notorious “Green Gap” in which LEDs are traditionally photon starved, ~500-600nm. The six sources can be turned on and off in any user-selected combination, and their output power can be independently adjusted over a 20-fold linear range (Figure 2). The light output of the Light Engine is extremely stable, with drifts of radiant and spectral intensity less than 1% over many hours of continuous operation.
The basic spectral output shown in Figure 2 can be reshaped in a matter of seconds under the control of the SPECTRA’s onboard microprocessor. This capability stands in marked contrast to the inflexible spectral output of xenon arc lamps. Xenon lamp output is spectrally continuous from 300 nm to 1200 nm and beyond. However, the output beyond 800 nm is generally not useful and can cause heat-induced tissue damage. In contrast, the SPECTRA Light Engine can be tailored to output only those wavelengths that generate useful light for visualization of the surgical field (Figure 2).
In practice, the SPECTRA’s five visible range sources (violet, blue, cyan, green and red, Figure 2) are used in combination to generate white light with correlated color temperature (CCT) and color rendering index (CRI) characteristics optimized for reflected light imaging of gross anatomy. The CCT and CRI characteristics can be adjusted and managed by the user via the Light Engine’s onboard microprocessor. The near-infrared output (nIR, Figure 2) is typically used for excitation of the fluorescent vascular contrast agent indocyanine green (ICG). Additional fluorescent contrast reagents such as protoporphyrin IX (PpIX) administered as its metabolic precursor 5-aminolevulinic acid (5-ALA) may be added if indicated for the type of surgery being undertaken.[3] Merging of fluorescence and white-light reflectance images provides enhanced anatomical context for surgeons performing complex microsurgical procedures.
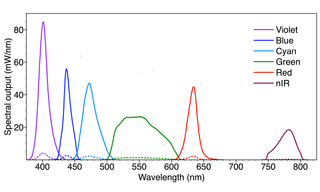
Figure 2. Spectral output of a SPECTRA Light Engine designed for use in a surgical exoscope. The output consists of 6 spectral bands generated by 6 independently controlled solid-state light sources. No light output is generated below 350 nm or above 850 nm. The sources in the 375–675 nm wavelength range are used in combination to generate white light. The power output of each source can be adjusted independently over a 20-fold range (minimum levels indicated by dashed lines) enabling optimization of the CCT and CRI characteristics. The invisible nIR output is used for excitation of the fluorescent vascular contrast agent indocyanine green (ICG).
Beyond its spectral output, the SPECTRA Light Engine offers three additional features that enhance its utility in intraoperative imaging applications:
1. Light delivery
The light output of the SPECTRA Light Engine is often delivered via an optical fiber bundle. The optical fiber bundle may be branched, providing two or more equally partitioned light outputs that can be directed to the surgical field at different angular trajectories. Multi-directional illumination circumvents accessibility and visibility restrictions, enabling three-dimensional imaging in confined spaces such as surgical fields.
2. Consistent inter-unit performance
This characteristic is vitally important in eliminating uncertainties in the installation and validation of the light source in an exoscope or other surgical device. Accordingly, the SPECTRA Light Engine is designed, manufactured and tested to deliver consistent performance from one unit to the next. Figure 3 provides a demonstration of this characteristic, comparing power outputs for 50 SPECTRA Light Engines manufactured to the same specifications as that represented in Figure 2. The standard deviations (n=50) in measured power outputs shown in the chart correspond to coefficients of variance (CV) of only 1–3%.
3. Built-in metrology and automation compatibility
Solid-state illuminators such as the SPECTRA Light Engine incorporate onboard microprocessors which monitor system performance in addition to controlling operations. Data such as cumulative light output duration, electrical power consumption and internal operating temperature are available for uploading to laboratory information management systems (LIMS) and for supporting regulatory compliance validation.
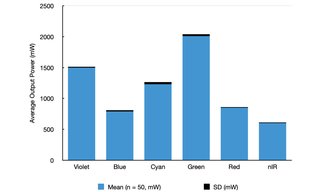
Figure 3. Maximum power outputs for each of the six solid-state light sources of 50 SPECTRA Light Engines manufactured to the same specification as that shown in Figure 2. The heights of the blue bars represent the average power output and the thicknesses of the black bars represent the standard deviations (SD) for the 50 samples. Total output for the six sources is 7 W per light engine, more than double the power output of a SPECTRA Light Engine configured for widefield fluorescence microscopy.
Improvements to visualization tools in demanding clinical procedures such as neurosurgery have fostered the use of exoscopes over conventional microscopes for enhanced surgeon ergonomics, comfort and patient outcomes. Exoscopes have the advantage of greater optical zoom, resolution, and illumination at a lower light intensity. Heads-up display for both the primary surgeon and other assistants permits neutral positioning of the surgeons while placing the camera in more angled positions. Such enhancements to surgical hardware demand state-of-the-art illumination which solid-state lamp replacements support well with consistent, long-lived, stable optical outputs. Spectrally tailored to meet the visible and nIR demands of clinical applications Light Engines shed durable, robust lighting, well-matched to traditional xenon bulb imaging yet optimized for the fluorescent dyes surgeons need for in situ visualization. Light Engines based on integrated solid-state sources are rapidly bringing about obsolescence of traditional bulbs and arc lamps for endoscopes, exoscopes, robotic surgery and a host of other deserving and demanding clinical applications.
- Jul 23, 2024
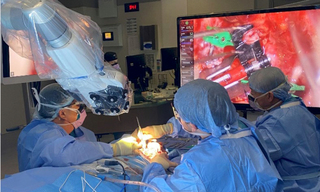
- (1) Johnson (2017) BioOptics World 52:3(opens in new window)
- (2) AJ Schupper, R Eskandari, CG Hadjipanayis et al. (2023) World Neurosurg 173:e571–e577(opens in new window)
- (3) N Montemurro, A Scerrati, G Trevisi et al. (2022) J Clin Med 11:223(opens in new window)
- (4) O Bin-Alamer, H Abou-Al-Shaar, CG Hadjipanayis et al. (2023) Cancers (Basel) 15:4890(opens in new window)